Technological advancements such as automation, robotics and artificial intelligence are driving the fourth industrial revolution. These same principles and technologies are finding their way into clinical practice and have the potential to revolutionise patient care.
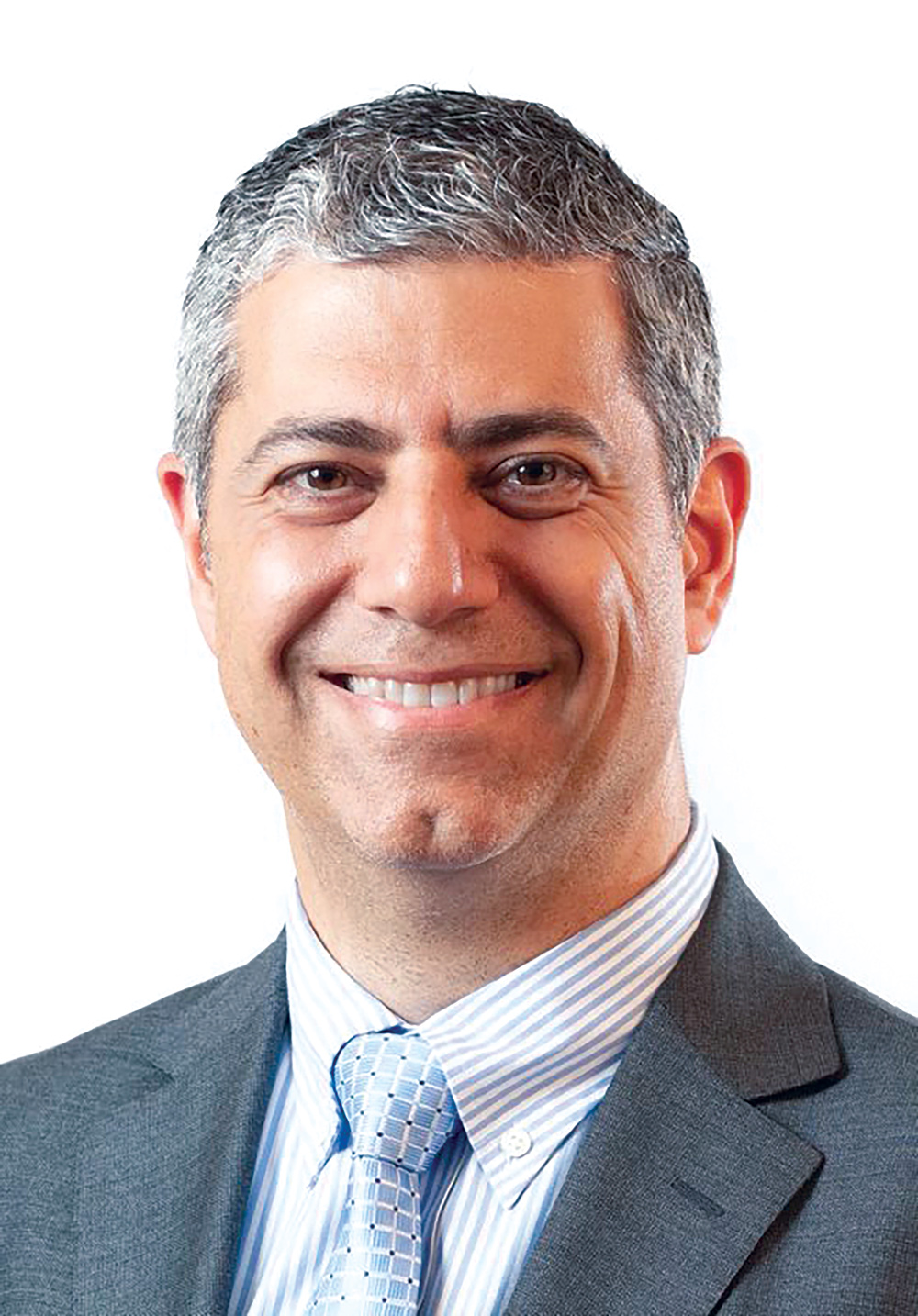
A technology that has broad clinical application in medicine, surgery and pathology is real-time in vivo endomicroscopy, a minimally invasive technique for obtaining histology-like images from inside the body, also known as optical biopsy. In endoscopic terms, it replaces the camera with a microscope.
One such platform is based on miniaturised confocal laser endomicroscopy (CLE) the size of a pen, producing in vivo high resolution digital images of cellular and sub-cellular structures at a thousand times real magnification without the need for tissue biopsy or laboratory processing, providing diagnostic information in real-time at the point of care.
Embedded within the tip of the miniaturised probe is a mechanical scanner, comprising a pair of lenses that focuses blue laser light (488nm) up to a depth of 800µm (Fig 1).
Fibre optic-based confocal endomicroscopes fall into two broad categories based on the position of the scanner compared to the light guide (either proximal or distal) and the composition of the fibre optic probe (either single or bundle), each with distinct advantages and disadvantages.
Single-fibre distal scanning endomicroscopes (so called endoscope-based eCLE) offer higher image quality and resolution with variable focal depth allowing imaging over a wider range, while bundle-fibre proximal scanning endomicroscopes (so called probed-based pCLE) benefit from their smaller size.
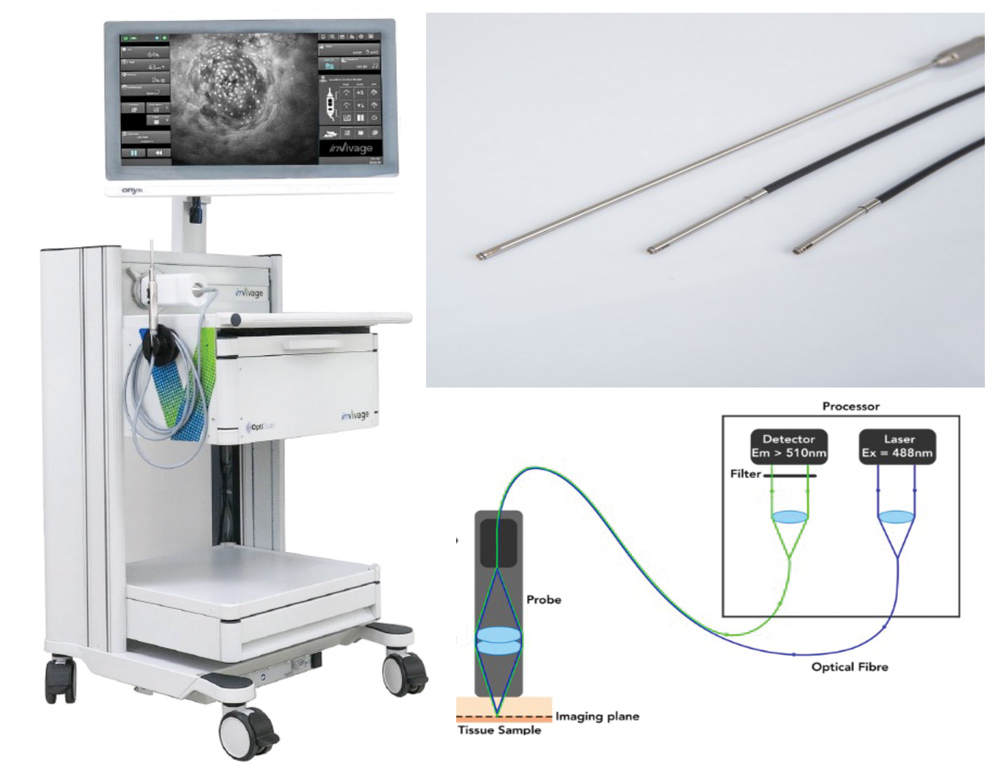
Confocal imaging can be based on tissue reflectance or fluorescence. Fluorescence-based endomicroscopes can be used with a variety of topical or intravenous contrast dyes, and can image cervix, colon, brain, breast, lung, kidney, liver, eye, oesophagus, muscle, tendon, and oral cavity amongst others (Fig 2). The platform can be used for pathological diagnosis, surveillance of disease processes, monitoring of drug distribution, and surgical margin clearance.
Commercially available CLE platforms can generate PACS-compliant DICOM 3D datasets which are streamable over local or remote networks enabling off-site assessment and truly integrated digital pathology workflows between surgeon and pathologist. 3D video reconstructions enable better understanding of disease processes.
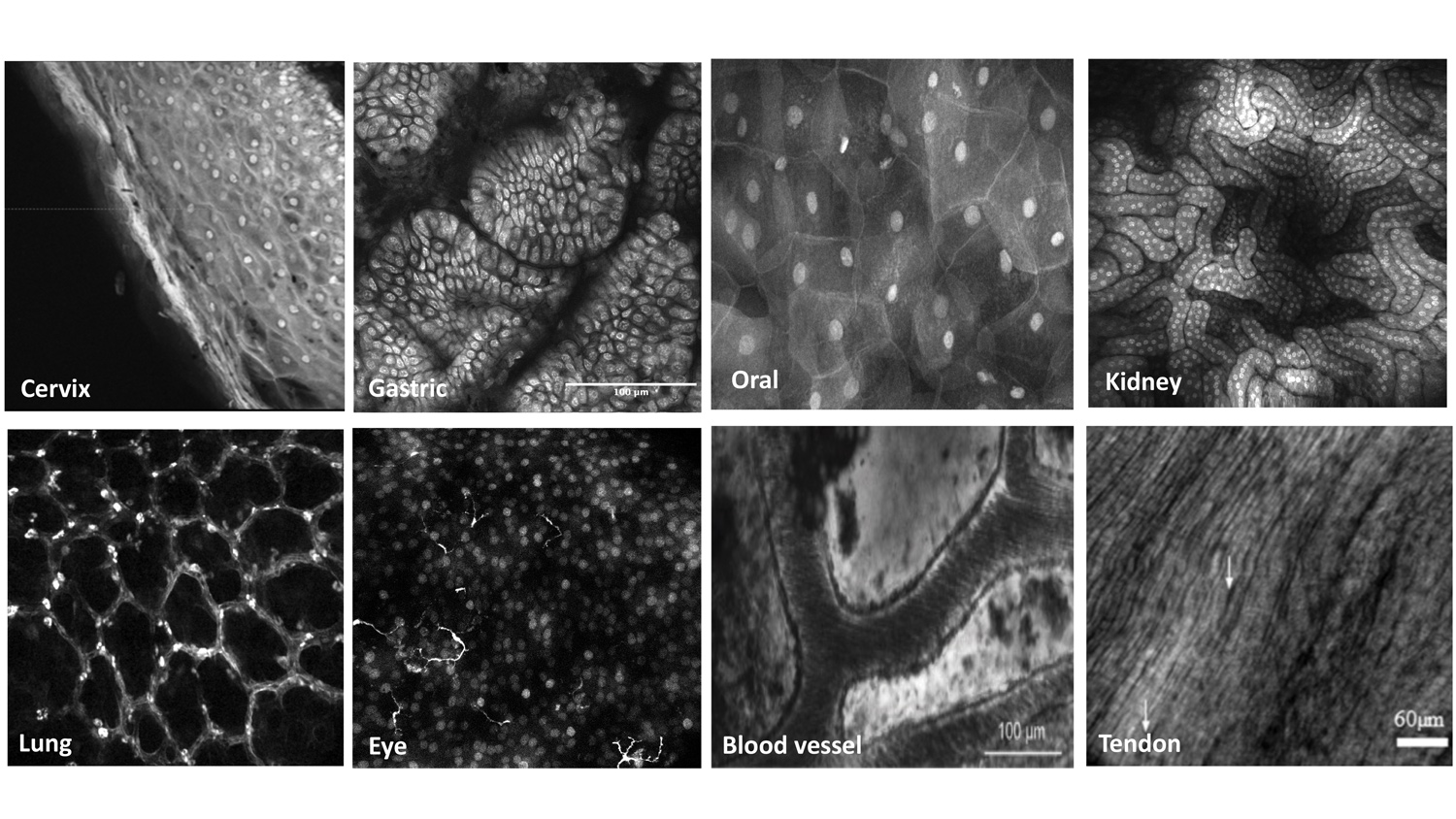
Likewise, the platform can be used in outpatient clinics for cutaneous or mucosal lesion assessment, or for inpatient procedures such as endoscopy, colonoscopy, cystoscopy and bronchoscopy, given the miniaturised lenses and scanners found at the distal tip of probes. These can easily access endoscopic working channels. In essence, the platform brings together the strengths of diagnostic imaging and tissue histopathology in the palm of one’s hand.
The technology has been assessed in a wide variety of human tissues and can easily differentiate normal tissue from cancer and pre-cancer (Fig 3), but also has wider applications in pharmacotherapeutics, vascular anomalies, and studying the microbiome.
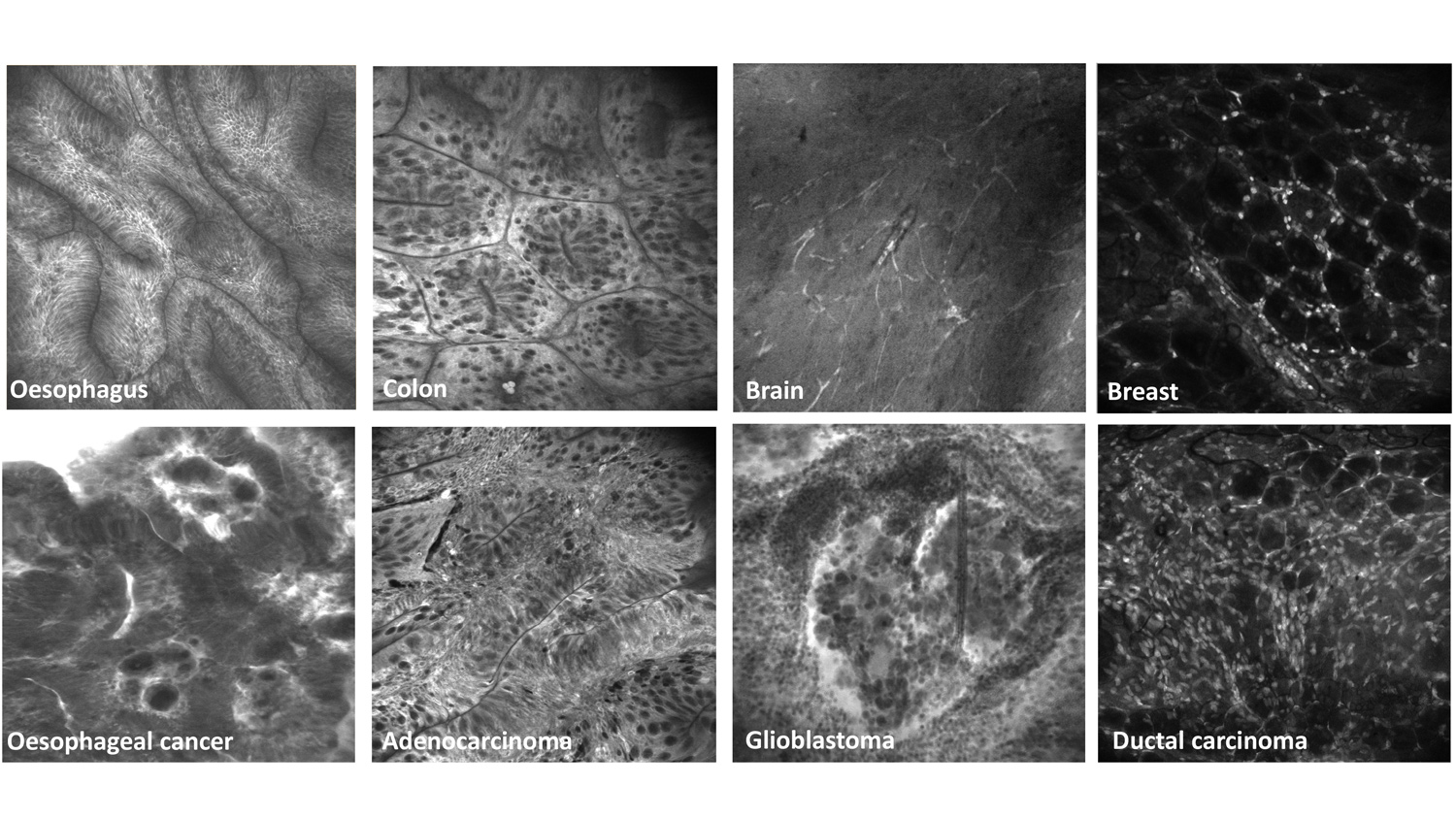
In oncological surgery, it can detect residual disease and more accurately determine clear surgical margins and has the potential to minimise adverse effects of adjuvant therapies. Images are comparable to traditional histopathology enabling visualisation of architectural, cellular, and subcellular features including cellular crowding, nuclear and cellular pleomorphism, and altered epithelial stratification in a manner similar to standard histopathological examination (Fig 4).
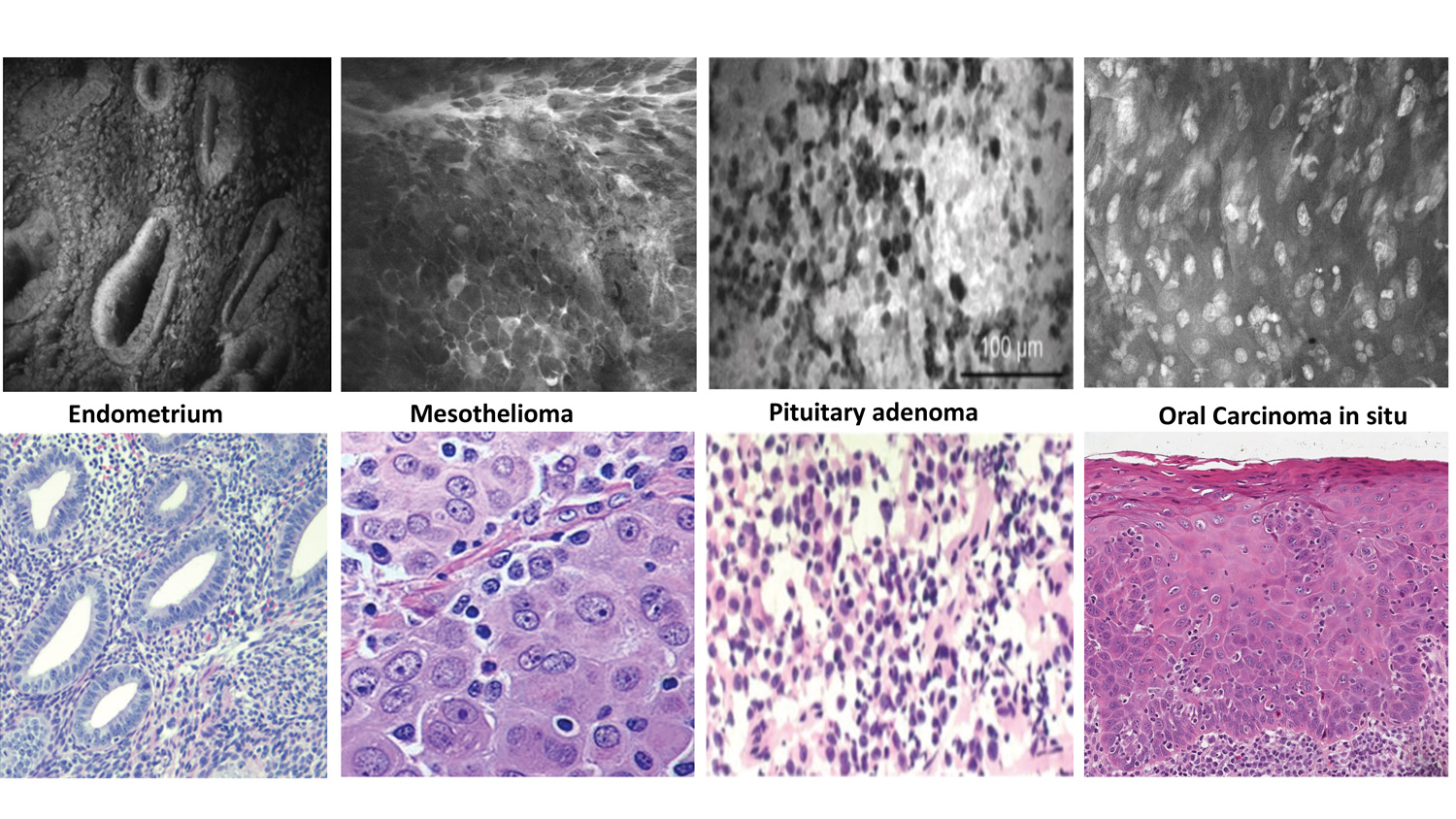
Coupling the technology with fluorescence-tagged specific disease biomarkers, it has the potential to detect the presence of lesions and determine their surgical clearance, enabling live molecular imaging at the cellular level, and bring us closer to patient-centred precision medicine.
Future applications include image segmentation, machine learning and artificial intelligence to generate quantitative readouts of pathological processes in a manner similar to that seen in automated radiological, cytological, and histopathological data-driven approaches. Potential applications are only limited by the user’s imagination and the clinical need.
– References available on request
Author competing interests – the author is Managing Director of Optiscan Imaging Limited (ASX: OIL), an Australian-based developer, manufacturer, and vendor of single-fibre distal-scanning fluorescence confocal endomicroscopes for clinical and research applications.